Antimicrobial Peptide
Reflecting recent work in the D'Amelio Lab
As antibiotic resistance surges worldwide, the search for effective new therapeutics has led scientists to a surprising source: the venom of Bungarus fasciatus, the banded krait. From this venom, researchers have isolated Cathelicidin-BF, CatBF, a cationic peptide that not only shows extraordinary activity against multidrug-resistant bacteria but also exhibits low toxicity and a favorable pharmacological profile. In a study published in the Journal of the American Chemical Society, researchers from the D'Amelio Lab at Université de Picardie Jules Verne, in Amiens, France, systematically evaluate CatBF’s antimicrobial action across clinical strains and explore its unique membrane-targeting mechanism using NMR spectroscopy and molecular dynamics simulations.
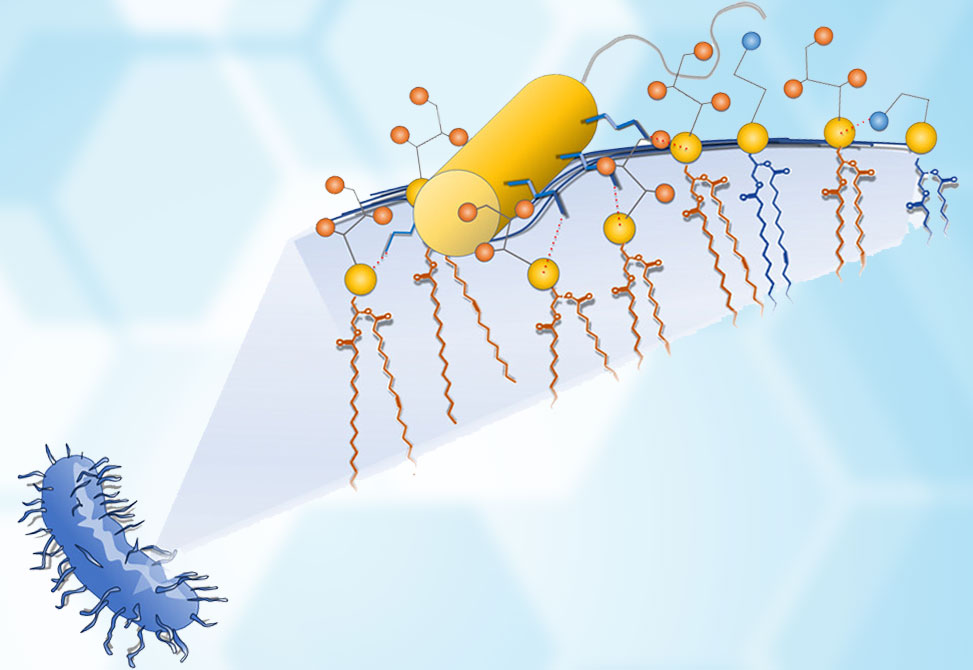
CatBF’s activity was tested against 81 clinical bacterial isolates, including highly resistant strains of Acinetobacter baumannii, Escherichia coli, and Klebsiella pneumoniae, many of which carried carbapenemases and other drug resistance mechanisms. Remarkably, CatBF exhibited submicromolar MIC values against a majority of these strains, with most isolates inhibited at concentrations between 0.5 and 1.1 μM. Its efficacy appeared unrelated to Gram classification or resistance genotype, suggesting a mechanism of action that bypasses traditional antibiotic targets entirely.
This hypothesis was borne out through structural and functional analysis. In aqueous solution, CatBF remains largely unstructured, but upon encountering model membranes—ranging from micelles and bicelles to uniaxially aligned bilayers—the peptide’s N-terminal region adopts a well-defined α-helical structure. NMR spectroscopy confirmed that this helix orients itself nearly parallel to the membrane surface, with its positively charged side chains likely establishing salt bridges with phospholipid phosphate moieties and its hydrophobic face making contacts with the membrane core. The C-terminal segment, beginning with a proline residue, remains flexible and unstructured—a design that may aid in versatility without compromising function.
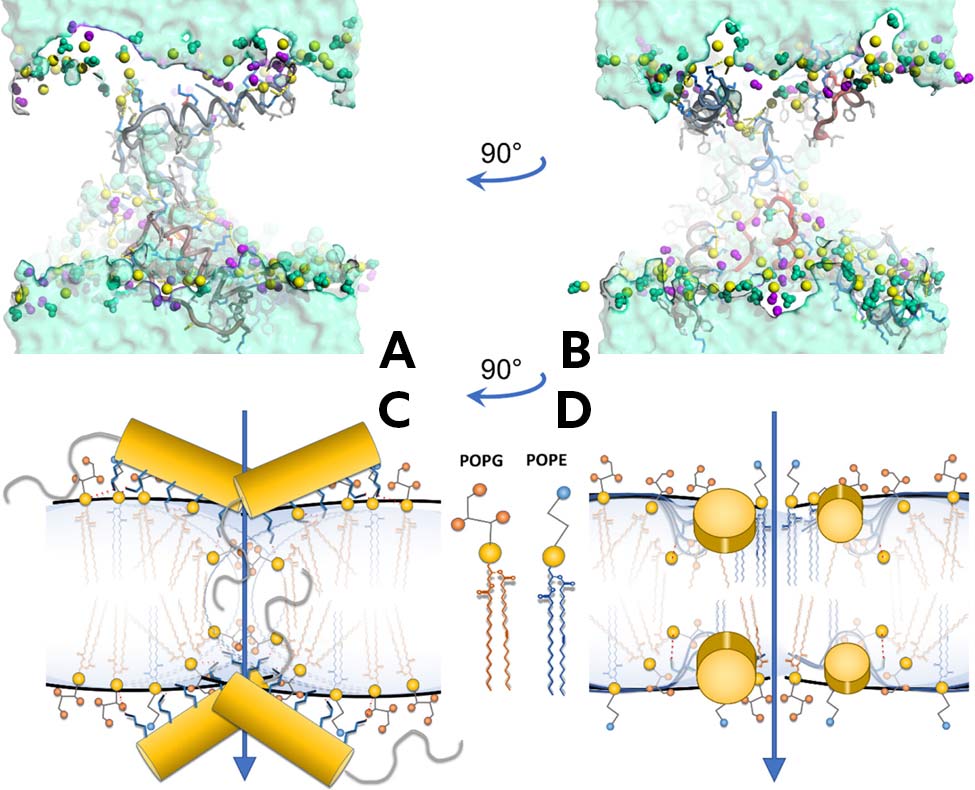
A-B: Representative MD snapshots displaying the formation of a toroidal-like pore in the presence of multiple CatBF peptides initially placed in the bilayer with their helices parallel to the POPE/POPG membrane surface. Color code: phosphorus atom: yellow; POPE dark green, body, turquoise, headgroup, light green, amine of the headgroup; POPG dark violet, body, violet, headgroup, light violet, hydroxyls of the headgroup. For clarity, only functional groups of lipid headgroups are shown as spheres). The CatBF peptide is shown as a “tube.” Side chains are shown as sticks with the following color code: positively charged, blue, negatively charged, red, nonpolar, light gray, and polar, yellow. C-D: A potential pore-formation mechanism in which the helices of CatBF, yellow cylinders, maintain an orientation nearly parallel to the membrane surface. Salt bridges between charged lysine/arginine residues, blue sticks, and lipid phosphate, yellow spheres, are shown as red dotted lines.
At low concentrations, CatBF inserts shallowly into bacterial membranes, positioning itself just beneath the phosphate headgroups. This strategic placement, combined with its high positive charge, +11, enables CatBF to rapidly neutralize membrane surface charge and destabilize lipid packing. Solid-state NMR and molecular dynamics simulations revealed that even a few peptide molecules can generate significant membrane curvature and perturbation—ultimately leading to the formation of toroidal pores. These transient, disordered channels allow for ion leakage and cell death, yet do not require the peptide to span the membrane fully or adopt a transmembrane orientation.
Interestingly, CatBF shows a strong preference for negatively charged lipids such as phosphatidylglycerol, PG, and cardiolipin, CL, which are enriched in bacterial membranes but rare in mammalian cells. This selective affinity helps explain both the peptide’s potent antibacterial activity and its minimal interaction with phosphatidylcholine-rich mammalian membranes, which remained structurally intact even in the presence of high peptide concentrations. Unlike many AMPs that must accumulate to high densities before exerting effects, CatBF disrupts bacterial membranes at very low molar ratios—a feature attributed to both its high charge and efficient surface recruitment of specific lipids.
As the peptide concentration increases, CatBF appears to self-associate on the membrane surface, producing cooperative effects that further destabilize lipid bilayers. At higher concentrations, changes in the peptide’s orientation and oligomeric state become apparent, suggesting a continuum of action that ranges from surface interaction and lipid demixing to pore formation and full-scale membrane collapse. Despite its potent activity, CatBF avoids nonspecific toxicity by sparing mammalian membrane models and limiting its depth of insertion.
Beyond its antibacterial action, CatBF’s structure and behavior suggest potential applications in antifungal and anticancer therapy as well. In simulations with phosphatidylserine-rich membranes—resembling those of fungi and apoptotic cancer cells—CatBF maintained a strong preference for interaction, forming hydrogen bonds and salt bridges while causing local membrane deformation. These interactions likely contribute to its observed effects in earlier studies targeting Candida species and tumor cells.
Taken together, these findings position Cathelicidin-BF as an extraordinarily promising lead compound in the fight against antimicrobial resistance. Its combination of broad-spectrum efficacy, resistance-agnostic mechanism, and selective membrane targeting offers a compelling template for rational AMP design. As global efforts intensify to develop novel antimicrobials, CatBF provides a blueprint—not only for how peptides can be engineered to overcome resistance—but for how nature’s molecules might hold the key to our most urgent clinical challenges.
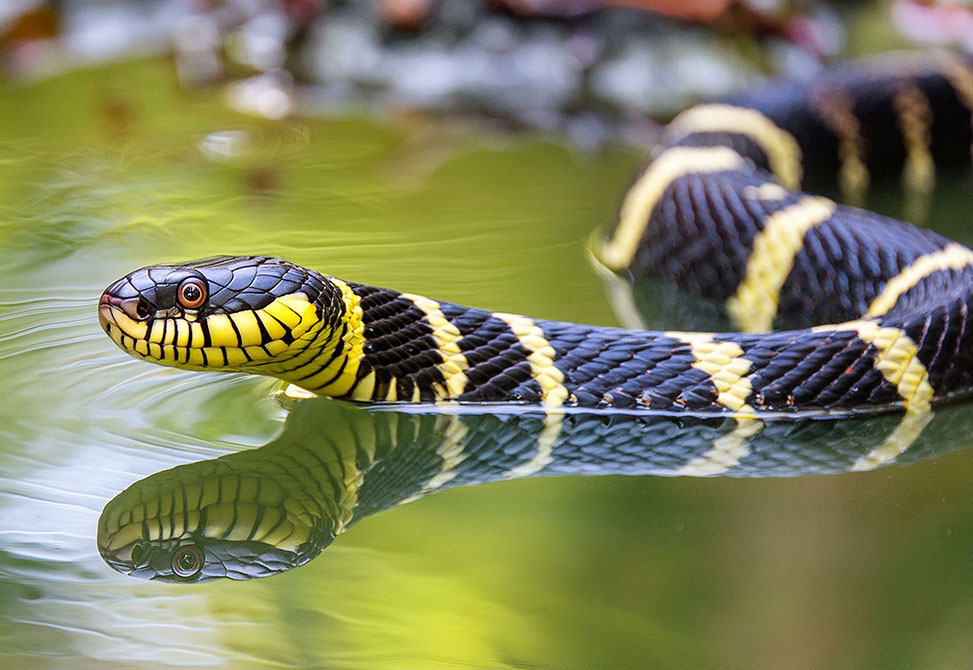