Arginine Carbonylation
Reflecting recent work in the Raj Lab
The selective incorporation of post-translational modifications, PTMs, into proteins remains a key challenge in chemical biology, particularly for modifications like arginine carbonylation, which plays a crucial role in oxidative stress and disease pathogenesis. Researchers at Emory University, led by Monika Raj, have developed a novel chemical carbonylation strategy that selectively converts arginine residues into glutamate-5-semialdehyde, enabling precise modifications of peptides and proteins. Published in the Journal of the American Chemical Society, this breakthrough provides the first non-enzymatic method for selectively inducing arginine oxidation, a modification previously limited to uncontrolled reactive oxygen species, ROS-driven, processes or metal-catalyzed oxidation, MCO.
Arginine carbonylation plays a key role in disease-associated oxidative stress, with implications in Alzheimer’s disease, cancer, diabetes, fibrosis, and chronic kidney disease. However, until now, no selective chemical approach existed for incorporating this PTM into proteins, limiting the ability to study its biological significance. Inspired by MCO mechanisms, the research team developed a metal-free strategy using 9,10-phenanthrenequinone derivatives, enabling direct conversion of arginine into glutamate-5-semialdehyde under mild conditions. This method provides a rapid, robust, and chemoselective approach to introducing this modification in proteins, facilitating chemoproteomic studies and offering a new tool for functional proteomics.
Key Advances in Chemical Carbonylation
Arginine’s guanidinium group presents a formidable challenge for chemical modification due to its low nucleophilicity and resistance to oxidation. The authors addressed this by leveraging diketone electrophiles, specifically 9,10-phenanthrenequinone analogs, which enhance conjugation and electrophilic activation, allowing for controlled oxidation of arginine. Through extensive optimization, they established reaction conditions in which aqueous-phase oxidation successfully converted arginine residues in peptides and proteins to glutamate-5-semialdehyde under mild, biocompatible conditions.
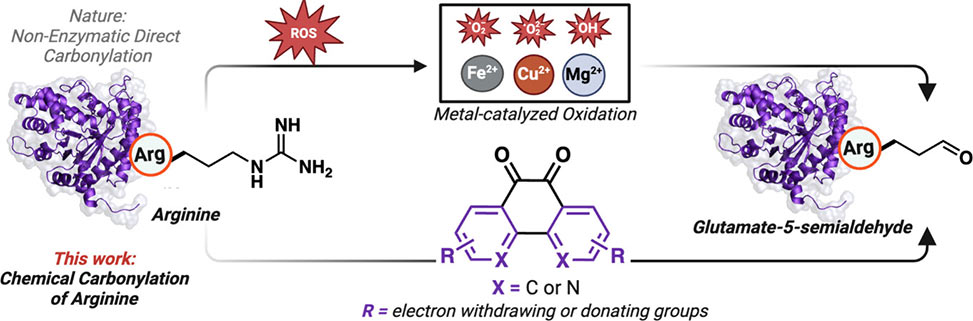
Figure 1. Platform for the selective carbonylation of arginine. Nonenzymatic oxidation of the guanidine group of arginine via metal-catalyzed oxidation, MCO, in the presence of ROS and reduced metal ions to generate glutamate-5-semialdehyde. This work: Selective carbonylation of arginine to generate glutamate-5-semialdehyde through the use of a metal-free chemical approach utilizing 9,10-phenanthrenequinone analogs. Created with BioRender.com, released under a Creative Commons Attribution-NonCommercial-NoDerivs 4.0 International license, Agreement number: TU27EWWM6M.
The method demonstrated high selectivity for arginine, with no cross-reactivity observed with other amino acids, including lysine, cysteine, and methionine, which are typically susceptible to oxidation. The team optimized the reaction using small peptides, and subsequently extended the strategy to full-length proteins, including myoglobin, aprotinin, ubiquitin, and RNase, achieving conversion efficiencies ranging from 70–95%.
In a proteome-wide chemoproteomic analysis, the method was further validated in cell lysates, revealing previously unknown arginine oxidation hotspots. By selectively modifying arginine residues and profiling them through mass spectrometry-based proteomics, the authors identified protein motifs enriched in carbonylation sites, including sequences rich in glycine, proline, alanine, and glutamic acid. These findings suggest that certain protein microenvironments are inherently predisposed to oxidative modification, providing insight into oxidative stress regulation and protein aging mechanisms.
Applications in Chemical Biology and Proteomics
Beyond its immediate implications for oxidative stress research, this chemical carbonylation approach serves as a versatile tool for protein modification, enabling:
Selective PTM Installation:
The aldehyde product of carbonylation serves as a chemical handle for further functionalization through reactions such as oxime formation and reductive amination. The authors demonstrated the site-specific incorporation of unnatural PTMs, including ornithine, methylated ornithine, and alkynylated ornithine, effectively expanding the scope of chemical protein editing.
Fluorescent Labeling of Carbonylated Proteins:
By leveraging aldehyde-specific fluorophores, the researchers successfully visualized carbonylated proteins in cell lysates and demonstrated selective in-gel fluorescence detection of oxidized proteins, providing a new method for live-cell imaging of oxidative modifications.
Cellular Profiling of Oxidative Stress Targets:
The study showed that chemically induced carbonylation could mimic oxidative stress conditions, offering a powerful platform for studying redox biology. The chemoproteomic analysis revealed that arginine residues in regulatory, enzymatic, and structural proteins are particularly susceptible to carbonylation, reinforcing the biological significance of this PTM.
Implications for Protein Carbonylation Research:
The ability to chemically install arginine carbonylation within proteins overcomes a major limitation in oxidative stress research. Previously, carbonylation could only be studied through indirect detection of oxidative damage, limiting the ability to precisely interrogate its role in protein function. This work establishes a direct, tunable chemical approach, providing researchers with a powerful tool for exploring disease-related protein oxidation.
Importantly, the method also sheds light on the structural consequences of arginine oxidation. The study found that carbonylation-induced Schiff base formation with lysine residues, potentially leading to protein cross-linking and aggregation, which are hallmarks of neurodegenerative disorders and cellular aging. The ability to generate and study these modifications in vitro provides a critical step forward in understanding how oxidative stress drives disease pathology.
Conclusion and Future Perspectives
This study represents a significant advance in chemical biology, offering the first selective, metal-free approach for arginine carbonylation. By successfully reproducing a biologically relevant PTM through chemical means, this work enables new research into protein oxidation, disease biomarkers, and redox-based cellular regulation.
Looking ahead, the authors highlight the potential applications in protein engineering, including synthetic protein design, molecular labeling, and drug discovery. Ongoing research in their lab is focused on adapting this methodology for in vivo studies, potentially opening new avenues for therapeutic interventions targeting oxidative stress-related diseases.
With implications spanning fundamental proteomics, chemical biology, and disease research, this breakthrough positions chemical carbonylation as a powerful tool for studying protein modifications at an unprecedented level of precision.
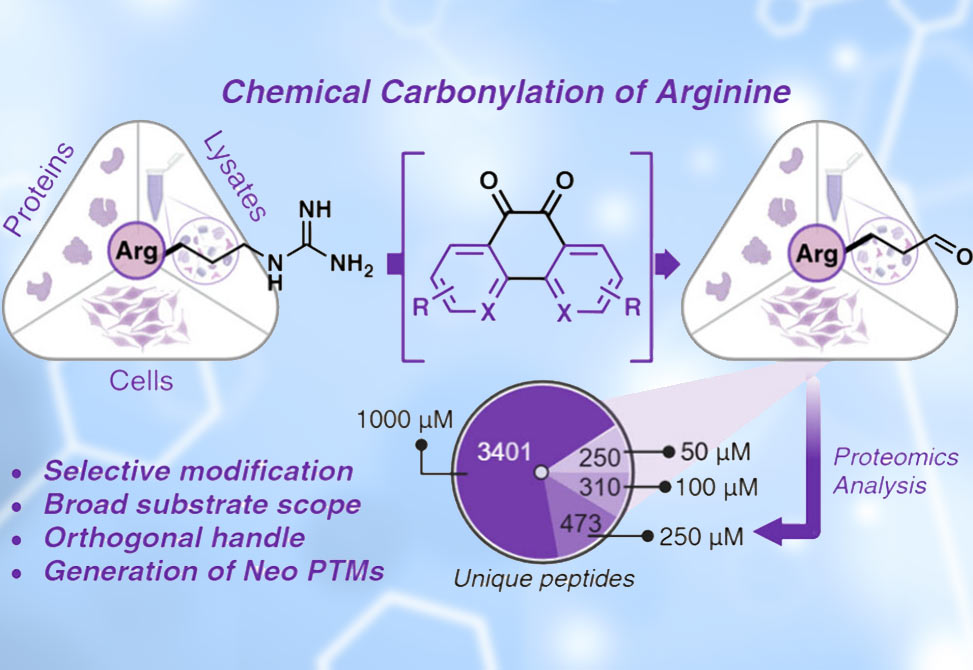