Backbone Editing
Reflecting recent work in the NSF CGEM
The ability to precisely manipulate the peptide backbone remains one of the fundamental challenges in biomolecular engineering. While ribosomal translation and peptide synthesis methods allow for modifications at the side chains, direct incorporation of carbon–carbon, C–C, bonds within the backbone has been an elusive goal. In a study published in the Journal of the American Chemical Society, researchers from the NSF Center for Genetically Encoded Materials, NSF CGEM, led by postdoctoral fellow Carly Schissel, present a novel approach to peptide backbone editing by leveraging a spontaneous acyl rearrangement mechanism, enabling the first nonenzymatic formation of C–C bonds within ribosomal and synthetically derived peptides. By harnessing the reactivity of dehydrolactic acid, DHL, the researchers demonstrate a cascade of acyl shifts that result in backbone-embedded, polyketide-like, α,γ-diketoamide motifs, which can be further diversified into heterocyclic structures.
Challenges in C–C Bond Formation in Ribosomal Products
Ribosomes are highly optimized molecular machines that catalyze the formation of amide bonds between α-amino acids. However, their synthetic scope has largely remained restricted to C–N, C–O, and C–S bond formations. Despite efforts to expand the ribosomal substrate range, the controlled introduction of backbone ketones—highly reactive motifs that enable late-stage functionalization—has remained challenging. Existing approaches to peptide backbone modification typically rely on side chain functionalization or post-synthetic chemical transformations. While direct, ribosome-mediated C–C bond formation within the peptide backbone remains elusive, our strategy leverages a post-translational transformation to achieve site-selective backbone modification, offering a programmable route to incorporate these key structural motifs.
A Novel Acyl Shift Cascade Enables Backbone C–C Bond Formation
This work introduces a strategy in which DHL-containing peptides undergo spontaneous isomerization to form α,γ-diketoamides, establishing a new C–C bond within the backbone. To enable this transformation, the α-hydroxy-phenylselenocysteine residue –an α-hydroxy acid derivative– can be introduced either ribosomally or via solid-phase peptide synthesis, SPPS. Oxidation generates DHL, and physiological conditions trigger an O to C acyl shift that results in diketone formation. The researchers demonstrate that these diketones are highly reactive and can be further derivatized using hydrazines and hydroxylamines to generate pyrazoles and oximes.
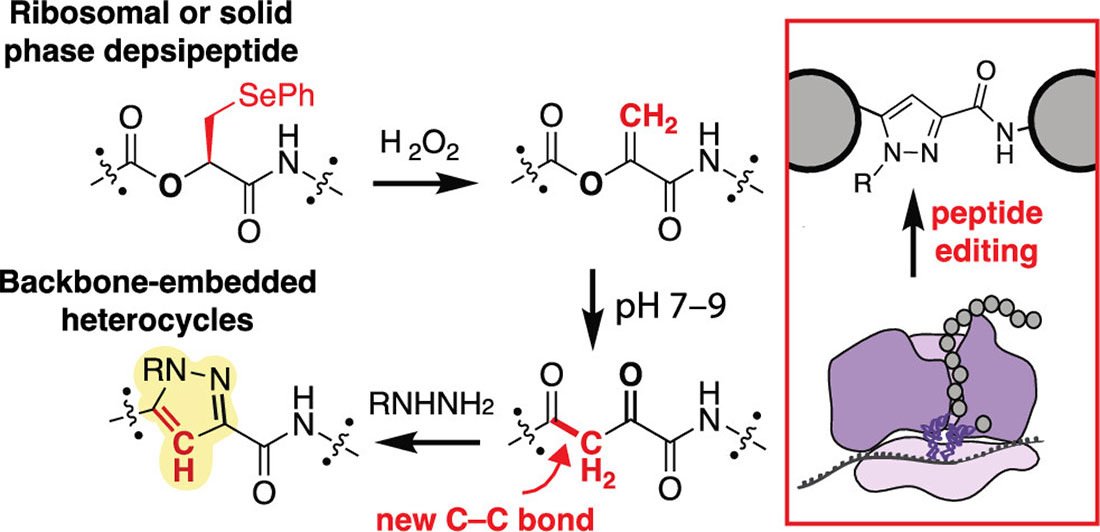
Mechanistic Insights and Computational Validation
Computational studies, done in collaboration with the Francis Lab, using density functional theory, DFT, reveal the thermodynamic feasibility of this transformation. The key C–C bond formation step is driven by the unique electronic properties of the DHL enolate intermediate, which undergoes an intramolecular cyclization. This reaction is conceptually similar to the Claisen condensation but takes place under physiological conditions without enzymatic assistance. The study also highlights the influence of pH on reaction efficiency, with optimal diketone formation occurring at neutral to slightly basic conditions.
Versatility and Synthetic Applications
The authors demonstrate the broad applicability of this method by incorporating DHL into various peptide sequences. Tripeptide and longer peptide backbones modified with DHL readily undergo diketone formation and subsequent derivatization, indicating that this transformation is compatible with diverse amino acid contexts. The study further extends this approach to ribosomally synthesized peptides, showing that DHL can be genetically encoded and subsequently converted into diketone-bearing peptides via post-translational modification.
Implications for Peptide Engineering and Drug Development
The approach presented by the researchers in the Schepartz lab, represents a significant step forward in peptide engineering, providing a new tool for embedding noncanonical motifs directly within the backbone. The ability to site-selectively introduce ketone groups expands the chemical space accessible to genetically encoded peptides, bridging the gap between peptide and polyketide scaffolds. Such modifications are expected to enhance peptide stability, membrane permeability, and bioactivity—key factors in drug development.
By offering a genetically programmable route to backbone C–C bonds, this work lays the foundation for new classes of bioactive peptides with unprecedented structural and functional diversity. Future applications may include the development of peptide-based therapeutics with enhanced resistance to proteolysis and tailored binding properties for molecular recognition.
Funding Sources
First author, Carly Schissel, is a postdoctoral fellow with Berkeley’s Miller Institute for Basic Research in Science, and her research is funded by the NSF Center for Genetically Encoded Materials, of which Professor Schepartz is the director.
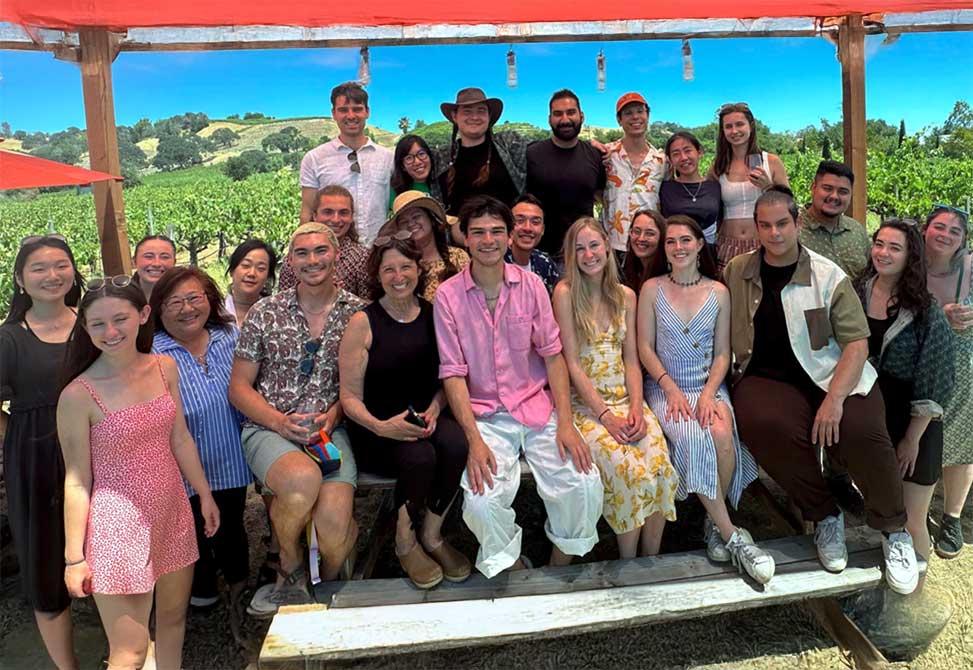