From Hydrogel to Crystal
Reflecting recent work in the Schneider Lab
Published in the Journal of the American Chemical Society, researchers in the lab of Dr. Joel P. Schneider at NIH, have unveiled a groundbreaking molecular design strategy that shifts gel-forming peptides into the crystalline state, revealing, for the first time, a high-resolution structure of a parallel rippled β-sheet — a long-theorized but previously unobserved motif predicted by Pauling and Corey over 70 years ago.
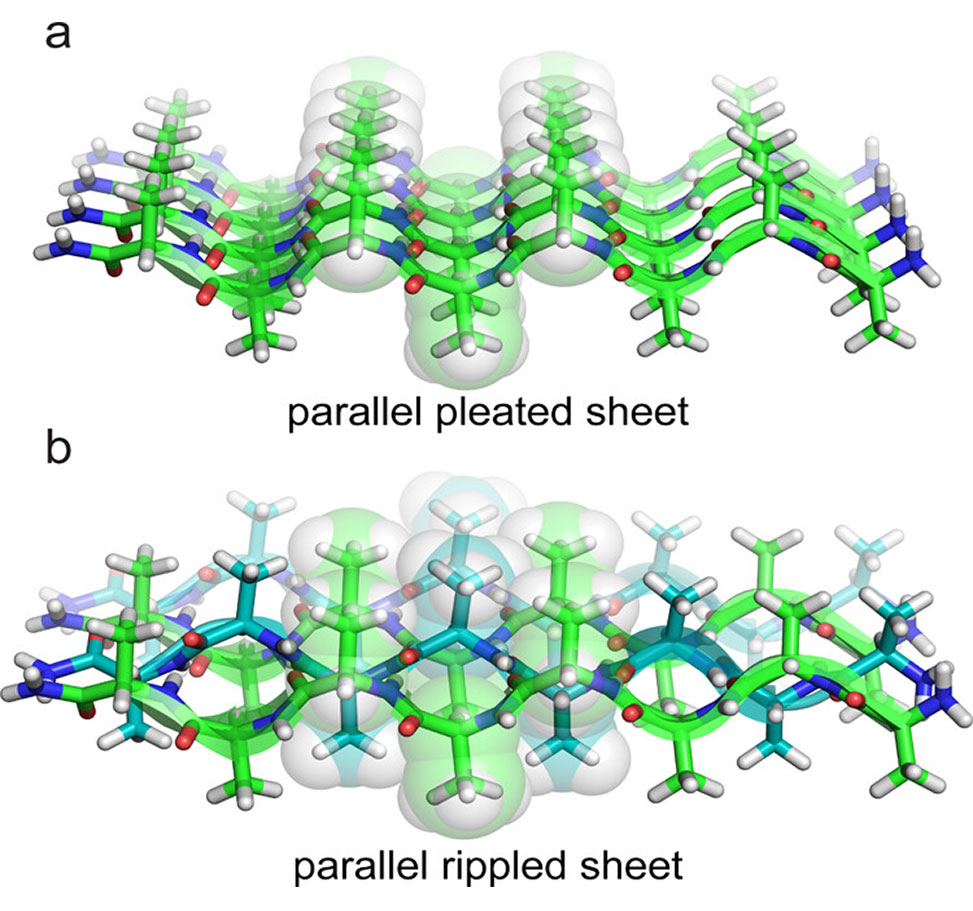
Figure 1. Polyalanine models of a (a) parallel pleated β-sheet formed between enantiopure strands of L-peptides, and a (b) rippled β-sheet formed between racemic mixtures of L-peptides, green, and D-peptides, cyan.
Self-assembling peptide hydrogels are central to biomaterials development, offering promising applications in drug delivery, tissue engineering, and immunomodulation. While racemic mixtures of L- and D-peptides have shown advantageous material properties — including enhanced stiffness, protease resistance, and thermal stability — the field has lacked atomic-level insights into the supramolecular interactions that govern these effects.
To overcome the crystallization challenges posed by flexible, fibrillar gel networks, the team developed a deliberate structure-guided approach: they truncated the parent MAX1 peptide, introduced cyclization via δ-linked ornithine, and incorporated Cα-methylation to guide and restrict hydrogen bonding. These modifications transformed the assembly behavior, yielding mirror-image macrocyclic peptides capable of forming crystals instead of gels.
High-resolution X-ray crystallography revealed that the racemic mixture of these cyclic peptides self-assembles into flat, fibril-like structures composed of alternating blocks of enantiopure β-hairpins. These domains form both canonical pleated β-sheets and — at the heterochiral interface — a parallel rippled β-sheet stabilized by interdigitated side chains and complementary van der Waals interactions. This ripple structure is framed by flanking Cα-methyl groups that define its registry and boundary — an elegant molecular bookend.
Importantly, these racemic fibrils were denser and more tightly packed than their homochiral counterparts, offering a plausible structural basis for the enhanced mechanical properties observed in racemic hydrogels. The findings not only validate long-standing theoretical predictions but also open a design pathway to engineer stereocomplexed hydrogels with atomic precision.
This work demonstrates that phase-state modulation through rational molecular design can serve as a powerful tool for revealing fundamental structural motifs. The identification of parallel rippled β-sheets provides a new scaffold for future biomaterials and invites the design of responsive, enantioselective peptide systems with built-in control over morphology, mechanics, and function.
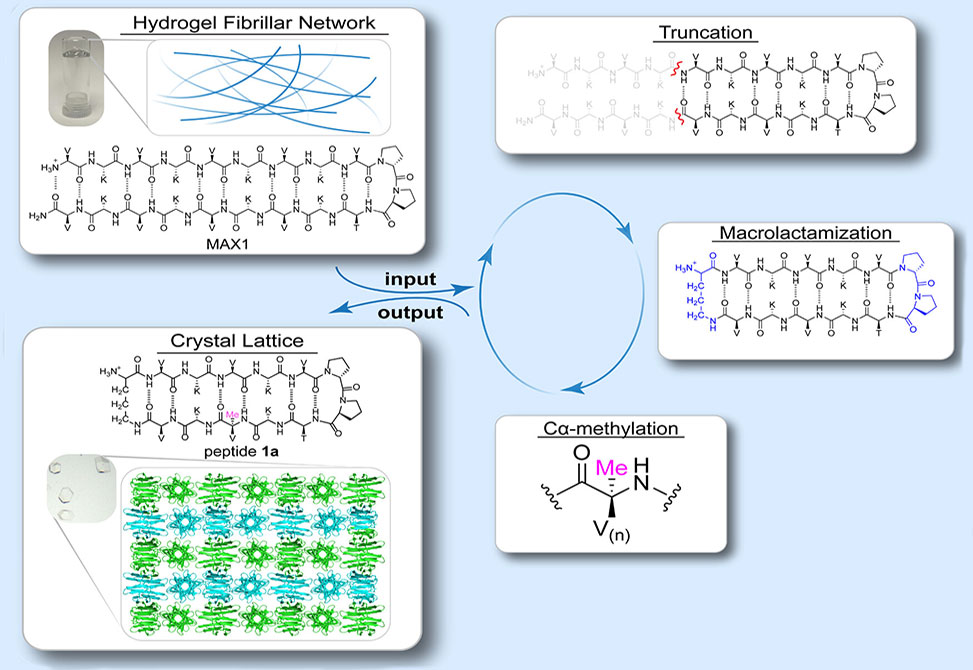